High energy fuels supply 80% of global energy, combined with cement processes, this accounts for emission of 30 billion tons of CO
2 annually.
1â3 This non-renewable source of energy is not only tethered to the global economy, but each day we rely on it we jeopardize the global climate. Implementing technologies that sequester atmospheric CO
2 and close the carbon cycle are paramount for stability of both the economy and the global climate. The electrochemical reduction of CO
2 (CO
2RR) is one promising technology to help solve this problem. However, the process of electrochemically reducing CO
2 is challenging, as driving selectivity to a given hydrocarbon product and mitigating the parasitic hydrogen evolution reaction (HER) both impede progress.
4 In the pursuit of more active and selective catalytic materials, researchers have been moving towards high surface area nanocatalysts and away from the planer Cu surfaces studied initially.
5 This transition results in a complex meso-scale catalyst layer that complicates diffusion of CO
2 to active sites. Evaluation of the intrinsic activity of new materials for CO
2RR is moot if CO
2 is not sufficiently being supplied to a catalystâs surface, especially when the electrolyte provides a near infinite supply of H
2O for HER.
6 In this report I will discuss my exploration into CO
2 electrolysis, from the development of highly active Cu NWs that displayed promising activity at low overpotentials only to disappoint at high overpotentials.
7 We later created a model of the electrolyte conditions within these nanowires and found supporting evidence that depletion of CO
2 at modest potentials (â-0.6 V vs RHE) was the likely cause of the apparent catalyst activity.
6Using this knowledge, I pursued alternative hierarchical Cu nanostructures that successfully demonstrated increased CO
2 consumption rates due to their lower active site density.
8Having learned the impact of meso-scale architecture and CO
2 diffusion, the nanocatalysts were adapted to a gas-diffusion electrolyzer, moving the bulk of CO
2 diffusion from liquid-phase (â2x10
-9 m
2/s) to gas-phase (â1.6x10
-5 m
2/s) to enable higher CO
2 flux. In this configuration we have been able to investigate physical structure-function relationships concerning catalyst layer thickness and utilization of catalyst active sites. However, findings demonstrate that the gas-diffusion electrolyzer can give a false sense of security about mass transport, as the catalyst layer thickness continued to play a major role in determining the output of the electrolyzer. Even under high CO
2 flux conditions there is an interplay between selectivity (Figure 1d), activity (Figure 1e) and catalyst layer thickness. The culmination of this work has most likely raised more questions than it has answered about ideal conditions in a CO
2 electrolyzer, but my future endeavors will continue pushing the limits of what is known, ultimately contributing to viable technological solutions that end reliance on non-renewable energy sources.
References
(1) Guo, Z.; Liu, B.; Zhang, Q.; Deng, W.; Wang, Y.; Yang, Y. Recent Advances in Heterogeneous Selective Oxidation Catalysis for Sustainable Chemistry. Chem. Soc. Rev. 2014, 43 (10), 3480â3524. https://doi.org/10.1039/C3CS60282F.
(2) Catalysts: - Specialty Chemicals Update Program (SCUP) | IHS Markit https://ihsmarkit.com/products/chemical-catalysts-petroleum-and-chemica… (accessed Apr 24, 2020).
(3) Global Carbon Project (GCP) https://www.globalcarbonproject.org/carbonbudget/ (accessed Apr 24, 2020).
(4) Verma, S.; Kim, B.; Jhong, H.-R. âMollyâ; Ma, S.; Kenis, P. J. A. A Gross-Margin Model for Defining Technoeconomic Benchmarks in the Electroreduction of CO2. ChemSusChem 2016, 9 (15), 1972â1979. https://doi.org/10.1002/cssc.201600394.
(5) Hori, Y.; Murata, A.; Takahashi, R. Formation of Hydrocarbons in the Electrochemical Reduction of Carbon-Dioxide at a Copper Electrode in Aqueous-Solution. J Chem Soc Farad T 1 1989, 85, 2309â2326. https://doi.org/10.1039/F19898502309.
(6) Raciti, D.; Mao, M.; Wang, C. Mass Transport Modelling for the Electroreduction of CO2 on Cu Nanowires. Nanotechnology 2018, 29 (4), 044001. https://doi.org/10.1088/1361-6528/aa9bd7.
(7) Raciti, D.; Livi, K. J.; Wang, C. Highly Dense Cu Nanowires for Low-Overpotential CO2 Reduction. Nano Lett. 2015, 15 (10), 6829â6835. https://doi.org/10.1021/acs.nanolett.5b03298.
(8) Raciti, D.; Wang, Y.; Park, J. H.; Wang, C. Three-Dimensional Hierarchical Copper-Based Nanostructures as Advanced Electrocatalysts for CO2 Reduction. ACS Appl. Energy Mater. 2018, 1 (6), 2392â2398. https://doi.org/10.1021/acsaem.8b00356.
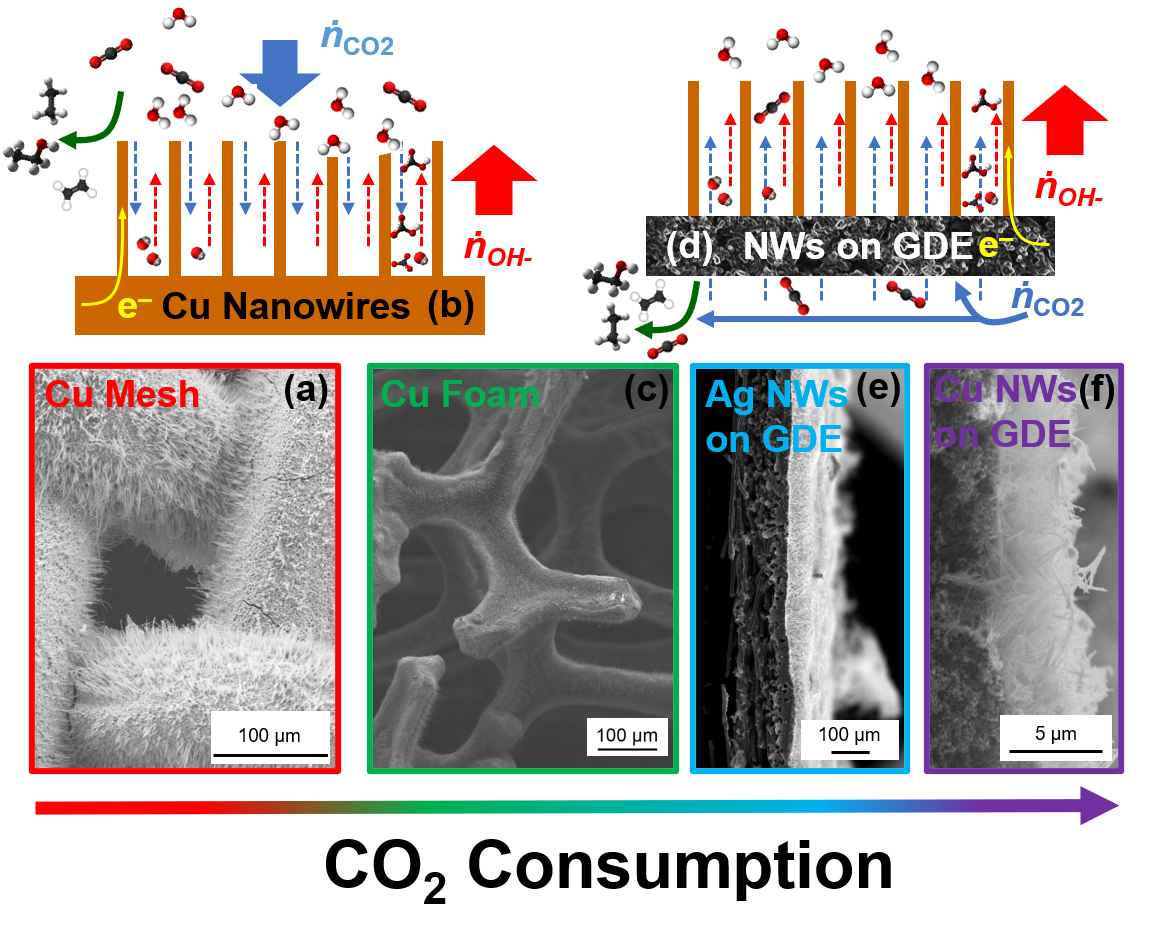